Podcast: Play in new window | Download
Subscribe: RSS
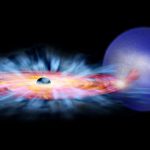
Black Hole Formation. Image credit: NASA
Why was there a difference between the amount of matter and antimatter at the beginning of the Universe? Mathematics lets us travel faster than light speed, so why can’t we? And are there stars forming around black holes?
If you’ve got a question for the Astronomy Cast team, please email it in to info@astronomycast.com and we’ll try to tackle it for a future show. Please include your location and a way to pronounce your name.
|
Shownotes
-
Why was there a difference between the amount of matter and antimatter at the beginning of the Universe? Do black holes have something to do with this?
- Why there’s more matter than antimatter in the Universe — Universe Today
- Discussion of the anti-matter black hole paradox — Astronomy forums
Mathematically, we can have a velocity greater than the speed of lights lets us travel faster than light speed, so why can’t we?
Can stars form in accretion disks around black holes?
- Accretions Disks –– NASA
- Cataclysmic variable stars — NASA
How much precision is needed to keep space telescopes focused on one spot? I have a hard enough time keeping my camera steady!
- How Hubble Works — HubbleSite
- How Gyroscopes Work for Hubble –– ESA
- Fine guidance sensors — HubbleSite
How can matter exist with antimatter?
- Antimatter- CERN
What causes sunspots and do they influence Earth in any way?
- Sunspots — Exploratorium
- Solar Flares — GSFC
- Coronal Mass Ejections — Cosmicopcia
How do we know what the speed of light is? How can we measure something that fast and how did scientists figure that out?
- Speed of Light , history of our knowledge about
- Ole Christensen Rømer
- Armand Fizeau
Would life as we know it be able to survive around a Red Dwarf?
- Living with a red dwarf — Astrobiology Magazine
- Tidally locked exoplanets — Martian Chronicles
Could matter escape from the event horizon of two black holes colliding?
- What happens when two black holes collide? — Universe Today
- No Naked Singularity After Black Hole Collision — Astroengine
- Intro to Black Holes
- Movies from the Edge of Space Time — UIUC
Why is it so bright in the center of the galaxy with a black hole there?
- The Milky Way’s Hidden Black Hole — Scientific American
- Sagittarius A*
What is behind a black hole?
- What is On the Other Side of a Black Hole? — Universe Today
- More about black holes — CWRU
Transcript: Questions Show: Matter Balance, Jumping Light Speed and Black Hole Star Formation
Fraser Cain: Welcome to the AstronomyCast questions show where we answer your questions about space and astronomy. If you have a question for the AstronomyCast team please email it in to info@astronomycast.com and we’ll try to tackle it for a future show. Please include your location and a way to pronounce your name.
Why was there a difference between the amount of matter and antimatter at the beginning of the universe? Mathematics lets us travel faster than light speed so why can’t we? Are there stars forming around black holes?
The first question comes from Scott Wells from Grand Forks, ND: “Is it possible that the slight imbalance in matter and antimatter was created not at the moment of the big bang but shortly after the universe formed by primordial black holes?”
That’s a complex question there. We’re talking about the slight imbalance at the beginning of the universe where all the matter and antimatter were generated and there was like one part per billion off and so they all collided, turned into energy but there was a little bit of matter left over and that’s what our whole universe is. Scott is asking could that matter actually be formed by primordial black holes. What?
Dr. Pamela Gay: This sort of falls into the let’s use black holes as a cure. [Laughter] They’re not. Black holes don’t care if you’re matter or antimatter. They eat you either way.
When they evaporate they give off matter and antimatter in equal parts. Black holes are kind of the ultimate neutral destruction device. They just destroy everything and then they spit out everything. They don’t care.
Fraser: Right, that’s kind of like that question that we had before. What if a black hole and an anti-black hole collide? You would just get a double the size black hole. [Laughter]
Pamela: Yeah you just get a lot of mass.
Fraser: Double the mass black hole, right.
Pamela: No, sadly we’re still stuck with for whatever reason this one part of physics is asymmetric and we have an asymmetric universe. It worked out for us.
If it wasn’t for that asymmetry everything would have annihilated and our universe would be pure energy and we wouldn’t have life. So we’re kind of glad for whatever went wrong in those beginning moments.
Fraser: Right. Shaun Crandall from San Antonio, TX asked: “According to special relativity we know that nothing with mass can reach the speed of light because the math and physics break down at velocity equals light speed. But at least mathematically it is quite simple to have a velocity of greater than the speed of light. Is it possible that you could jump over the velocity equals light speed and be able to move faster than the speed of light?”
Pamela: No.
Fraser: No. Even though the mathematics works out?
Pamela: Math allows lots of things. Math has no boundaries on it other than certain rules like 2 plus 2 must equal 4. Math isn’t constrained by physical reality. It doesn’t care that you have infinite mass. It doesn’t care that you’re dividing by zero. It simply is.
So while the math will let you have whatever velocity you want the reality of the universe says no c is the ultimate speed and you can’t go any faster than that. It’s very unsatisfying.
Fraser: It’s not that math and physics break down it’s that our understanding is what’s doing the [laughter] breaking down.
Pamela: In this case physics simply says you can’t go faster than the speed of light and the math will let you play but once you start looking at the mass you now have nonsense.
Fraser: Dawn Hoverson asked: “Since black holes can generate temperatures and pressures in their accretion discs is there an appreciable amount of nucleosynthesis taking place?”
I guess what Dawn is asking is around black holes can you have the accretion disc get hot enough and have high enough pressure that you get nucleosynthesis like we have at the core of our sun or even more hotter stars?
Pamela: Yeah and what’s really cool is you don’t even need a black hole. When white dwarfs get too close to a companion star in binary systems – we call this a cataclysmic variable because they’ll start gravitationally grabbing matter off of their companion star sucking it toward them and filling up an accretion disc. Periodically this accretion disc will fill up and undergo nuclear reactions.
We can end up with these nuclear reactions going on not just in the accretion discs of black holes but in the accretion discs of neutron stars and the accretion discs of white dwarfs. When you ask is there appreciable Nucleosynthesis that’s a kind of vague adjective.
It’s like asking have I drunk a lot of water today. I’m not sure. It depends on whose definition you use. There is a fair amount of nucleosynthesis in terms of given how long reactions take place and how much matter is involved. It’s kind of like dealing with a star and that’s kind of cool.
Fraser: Ronald Chan asked: “How much precision is needed to take those long exposures with a space telescope in orbit? I have enough trouble keeping a camera from moving around on Earth with a sturdy tripod and sandbags. How can you keep a space observatory pointed at a subject with enough precision to get a clear image?”
That’s absolutely true you may have Hubble staring at the same object for hours and hours and hours. In some cases with the deep field, it was hundreds of hours. How can you get it not even jiggling just a tiny little bit?
Pamela: It’s a combination of gyroscopes and more gyroscopes – well you can do it with two gyroscopes. They also use a fine guidance camera. The gyroscopes if you’ve ever taken a bicycle tire and held on to both sides of it while it’s not touching the ground and spun it and then tried to rotate it, it’s really hard. Things that are rotating are naturally stable.
On the Hubble space station they have a set of six different gyroscopes that can control the space station very precisely using any two of them. They also have a Fine Guidance camera. The Fine Guidance camera locks on a bright star and it stays centered.
The gyroscopes can be used to make fine corrections to the space telescope’s position. So, you’re constantly imaging using the Fine Guidance camera and you’re using the gyroscopes to keep yourself locked in position.
Fraser: Those gyroscopes actually resist the movement. It’s almost like you’re trying to push the telescope uphill away from where it wants to be to actually make it to move.
Pamela: These gyroscopes are floating in fluid and they actually use the fact that the gyroscopes don’t want to move to rotate the space telescope around the gyroscopes.
Fraser: That’s why you need at least two so you can move in two directions, right?
Pamela: Yes.
Fraser: Three is better.
Pamela: Three is better but they’ve figured out how to use things like the Earth’s magnetic field and other stuff to keep track of where we are in that third dimension.
Fraser: Right but without the gyroscopes those long images would be really hard to take.
Pamela: It would never happen.
Fraser: Okay, next question comes from Joshua Eagles from Australia: “How can matter exist? Shouldn’t we be annihilated by antimatter so that there is no overall net force? Are we in a small unfortunate part of the universe that may have large quantities of matter but over the universe as a whole there is no net value?”
I guess what Joshua is asking is is it really that the universe is all matter? You know after that original – we talked about early in the show – you have the matter, you have the antimatter, they collided and there was a little bit of matter left over and that’s why the whole universe is matter.
Is that the case? Or is it like pockets of matter here and pockets of antimatter over there and later on we might get overrun by a pocket of antimatter.
Pamela: As far as we know we actually live in a universe that is dominated by regular matter. While we do actually interact with antimatter all the time there are only certain reactions that are allowed.
For instance, antimatter neutrinos don’t react at all with normal matter electrons or protons. You probably have antimatter neutrinos of one variety or another flying through your body all the time and your body’s matter just doesn’t care.
You can have positrons and electrons, the antimatter version of the electron and the regular matter version of the electrons. They’ll instantly annihilate against each other.
Proton, antiproton – instantly annihilate against each other. There are only certain types of reactions that are allowed.
What antimatter is flying around all the time we don’t generally have to worry about too much? We live in a matter-dominated universe so we’re okay.
Fraser: We can see matter and antimatter collisions happening around the universe. It gives off very specific energy characteristics. If we looked up in the universe and we saw that kind of energy happening we would say oh wow there’s still lots of matter and antimatter colliding all around us. Get ready for a cloud of antimatter to come through the solar system.
We don’t see that at all. We see some places where there is matter and antimatter colliding but it’s more like it is being caused by something else. We see some around the center of the Milky Way.
Pamela: This is just a matter of different places are producing antimatter and the universe takes care of it for us. We’re okay.
Fraser: Paul Williams from Hong Kong asked: “What causes sunspots and does it influence our Earth in any way?”
Okay a sunspot is a black spot on the sun, what’s causing those?
Pamela: Those are actually places where the sun’s magnetic field has for one reason or another decided to poke itself up to the surface. The sunspots pretty much always appear in pairs.
One has basically a north magnetic pole and the other one has a south magnetic pole. You can imagine the magnetic field arcing between the two different locations on the surface of the sun.
They look dark but they’re actually just a thousandish degrees cooler than their surroundings. If you could see one of these sunspots all by its lonesome they are hotter than an arc welder.
Fraser: Right, they’re only 5,000 degrees Kelvin as opposed to almost 6,000 degrees Kelvin. [Laughter]
Pamela: Right. These are places where the energy has gotten all tangled up in the magnetic field. Periodically these magnetic field lines disconnect and reconnect in the process giving off huge amounts of energy.
They release vast amounts of plasma and high energy particles and high energy electrons all into the space around the sun. That stuff sometimes does head our way and create if we’re lucky, just nice northern lights, nice aurora that we can observe; southern lights.
Sometimes there is too much stuff and it can be dangerous for astronauts. It can wipe out satellites. If the magnetic field of the Earth gets whacked too hard the oscillating magnetic field can generate electric current in already overburdened power lines and take out the power grid.
That is a rather dramatic bad thing, so we generally try and keep an eye on the sun so that we can moderate where the astronauts are, what is the power on the power grid? All of this is in response to the sun’s activities.
Fraser: Right but the sunspots are often the sources of these flares. The coronal mass ejections so they don’t influence us temperature-wise or things like that.
Yes you sometimes get these flares and sometimes these flares give us a pretty light show. They influence our hearts. [Laughter]
John Painter from Millbrook, Ontario asked: “How do we know what the speed of light is? How is it possible to measure something going that fast? It seems as if scientists have known what the speed of light is for at least 100 years, so how did they discover it?”
Okay Pamela, so what is the speed of light?
Pamela: The speed of light is 299792.458 kilometers per second.
Fraser: Is that from memory?
Pamela: No. From memory it’s 300,000 kilometers per second.
Fraser: That’s fast. That’s here to the moon in a second.
Pamela: By definition we have actually defined a meter using and thus defined a kilometer as well, using the speed of light. By definition, the speed of light is 299792.458 kilometers per second. It’s fast.
Fraser: Right, that is fast. You can’t – Channel 9 okay and then I press a stopwatch [laughter] and then the light comes past me and I press the stopwatch again, because the speed of me seeing the light is the speed of light.
Even if I had a car going the speed of light I wouldn’t know to press start until it had just got past me. [Laughter] How on Earth, a hundred years ago did these people figure out the speed of light?
Pamela: It was actually more than a hundred years ago. People first started making measurements of the speed of light back around 1676.
Fraser: Wow.
Pamela: [Laughter] That’s kind of amazing to think about. First we needed the telescope but once we had the telescope it started to become practical.
I’m going to pronounce this poor soul’s name; someone whose name vaguely resembles Ole Rømer was looking at eclipses of the moons of Jupiter. What he noticed was if the moons were traveling toward us the eclipses lasted one amount of time and if they were moving away from us they lasted another amount of time.
He didn’t know the distance to Jupiter very precisely and thus didn’t know the sizes of the orbits very precisely. What he was able to figure out was that the light travel time would be longer if the object was moving in one direction than the other because it would have gotten further away by the time the eclipse ended or gotten closer by the time the eclipse ended.
This ends up changing the duration of the eclipse. He ran all the math and ended up with the speed of light at 214,000 kilometers per second which isn’t bad.
Fraser: That’s not bad at all.
Pamela: No, so that was simply based on looking at the moons of Jupiter. A more precise way is to instead use basically a spinning wheel and mirrors. The first particularly precise measurement that was made was by Armand Fizeau in 1849.
He took a mirror eight kilometers away from where he was planning to stand and had a wheel with teeth – think of a gear basically. He started it rotating and shined a light through it. If he had the rotation rate of the wheel just right, the light would go through the tooth and reflect right back through the next tooth.
He was able to essentially send a pulse of light and measure the pulse coming back. He was looking for basically the travel time for a ball of light which is something that you can figure out.
Fraser: His was very accurate, right?
Pamela: Yeah he was able to actually get 298,000 kilometers per second. He was able to get very precise.
Fraser: This was still a couple hundred years ago so this is an experiment that you could probably do in university? If you had enough careful manufacturing and set up the experiment it’s something that you could replicate, right?
Pamela: You don’t even need careful equipment. This is stuff that people were making prior to having big fancy machine shops. It’s possible to basically go out, buy yourself a nice big mirror, get yourself a chunk of wood and cut notches out of it and attach it to an axle and start it spinning very precisely.
You just need to have some sort of a mechanism to measure the frequency at which it is spinning. You can do that simply by counting how many times does it rotate per minute using an egg counter of some sort.
Fraser: I love it. This is one of the how do we know what we know questions. I think it’s great that people call us on it and ask how do we know and we can go back and look up the history and see the experiments and explain them.
Steve Davis from Rico, Australia asked: “I want to know if life as we know it would survive on a planet orbiting a red dwarf. With the advantage of a long life a red dwarf would seem like a logical place to find advanced life and if so, perhaps we should be listening to them with SETI.”
Red dwarf stars are stars with a fraction of the mass of our own sun. I guess since we have planets going around the sun and one of those planets happens to have life and it is likely there are planets going around a red dwarf.
Red dwarfs are thought to last hundreds of billions maybe even a trillion years. That might be the place where life could really get a chance to take time and evolve and become advanced. Are there any problems with that idea?
Pamela: Yeah, red dwarfs are very angry in their youth. These are stars that when they’re in the process of forming give off massive amounts of x-rays. That’s fine, stars do that no big deal but with a star like our own sun planets like Earth got to keep their distance.
We were a fair distance away from our angry young sun. The planets around red dwarfs that are going to be capable of eventually supporting life have to be just about on top of their parent star. They have to be in extremely close to get enough warmth to be able to have liquid water.
The angry youth of a red dwarf lasts about a million years. It is going to destroy any proto-life that starts to form on a planet that is close enough to have liquid water for those billion years of anger.
It is possible that a planet close to one of these red dwarfs could eventually form life or could be seeded with life from someplace else. We can’t rule them out.
The other thing we do have to worry about though is the planets are so close to their parent star that the probability that they get tidally locked, that just like our moon only one side of the planet ever faces its star is pretty great.
When you end up with tidally locked planets you end up with really weird, really harsh weather patterns. That would make life much more difficult to evolve. But, it’s non-zero. It’s just another place with another set of challenges.
Fraser: But I think a few years ago everyone was like no, there’s no point. It’s not hot enough or the stars are too violent. I know that even at the last couple of AAS meetings there were some really interesting discussions. I saw some neat poster presentations where people were talking about maybe it is not quite so violent.
Maybe you could have a planet move in from further away after the violent period and then settle into a nice orbit. I think there is some really interesting research that’s going on right now to answer that exact question.
You’re right, the implications are if it does seem that they are viable places for life there is lots more regular stars out there than there are stars like the sun. Some of them are very close.
They would make really good candidates for pointing your SETI telescopes out to listen for communications. I think that’s a really good path that scientists are considering.
Pamela: And at the end of the day what we’re finding is any type of a star pretty much can support planets. It’s just a matter of always looking everywhere.
Fraser: Peter Kajodik from Serbia asked: “Is it possible for matter or energy to escape from an event horizon during the collision of two black holes?”
Hmm I guess you’ve got normally, nothing no matter, nothing no light, no energy, no matter can ever escape from within the event horizon of a black hole. But if you have two black holes colliding could you get a scenario where something could escape and leak out?
Pamela: I have to admit I was doing back of the envelope force diagrams. From what I can figure out if you have in isolation two black holes that are in the process of merging, they’re spiraling and spiraling in toward each other. You will end up with things that had had an escape velocity that was speed of lightish and was thus inside of an event horizon.
Because you now have the gravitational pull of the two black holes that escape velocity will sink down so that it is lower and thus you could, if you were able to fire off rocket engines or something, escape. The problem is I couldn’t think of any way to easily induce the force necessary to get that sucker to escape.
You have things spiraling together, spiraling together in the event horizons will move. But I couldn’t figure out how to get something that had previously been inside an event horizon happily orbiting or at least as happily orbiting as you can happily orbit inside of event horizons.
Fraser: Terrified, leave orbiting. [Laughter]
Pamela: Right. I couldn’t figure out how to get any of these terrified orbiting objects to get a force to suck them out in a different direction.
So, yeah you can have something that suddenly is no longer within an event horizon but then I wasn’t sure how to get it out of the system.
Fraser: Right, I guess I don’t know, instinctively and I know when you’re talking about black holes and relativity it’s all pointless, but it feels like if you’ve got an event horizon for one and you have an event horizon for another and you put them together..
Pamela: What you end up with is..
Fraser: Something bigger with a bigger event horizon but it would still encompass both objects, am I right?
Pamela: No that actually, so back of the envelope again what you can imagine is one black hole in isolation ignoring spin is going to have a nice symmetrical event horizon around it.
Another black hole in isolation is going to have a nice spherical event horizon around it. If you imagine bringing these objects closer and closer together what you end up with is basically a flattened event horizon on the sides closer to the two objects because they’re both trying to yank from the other one the point at which you have to exceed the speed of light to escape ends up getting closer to the black hole on the side where the two are closest to one another.
It ends up going out in a teardrop shape away from the center of the two systems. You can imagine basically two teardrops coming toward each other trailing the droppy bits to the outsides.
As they get closer and closer the event horizons flatten more on the side that are facing each other until they reach the point that you end up with one event horizon around both objects. It’s complicated and then once you start adding motion in, it gets even more complicated.
Fraser: You’re saying then that maybe it is theoretically a way you could extract something back out of a black hole. Hit it with a bigger black hole.
Pamela: Well, the problem is then where you get the extra force that gets the thing to come out and a third direction going.
Fraser: Right so it has to be able to use energy then to get out. You’re not going to get it just from the collision itself.
You would have to have something with an antimatter drive that is going to try and push at the right moment [laughter] to take advantage of this unique situation.
Pamela: Or something. The fact of the matter is in general, once something is inside the event horizon it is spiraling towards its death.
Bringing two black holes together you’re going to muck up the distribution of the event horizons and it is possible you’ll move one in.
It’s possible you can yank something around somehow but setting it completely free of the system – no. Not without extra forces.
Fraser: Justin Song from Chicago, IL asked: “Why is it so bright in the centers of galaxies if there are supermassive black holes that suck up that light?”
I know we’ve answered this question in various flavors before but this is an easy one, we’ll tackle it. So Pamela, there’s a black hole at the center of a galaxy, why is the center of a galaxy bright?
Pamela: Because there’s stuff in there other than the black hole that gives off light.
Fraser: Right, stuff waiting to die; stuff being mushed around the black hole.
Pamela: Stuff waiting to die. Even in our own Milky Way as you look towards Sag A stars – you look towards the constellation Sagittarius through all the dust and gunk between here and the center of the galaxy. The inner part of the galaxy is really dense with stars.
It’s that density of swarming stars that in normal galaxies makes the quark quite bright. In quasars in active galactic nuclei that center is even brighter because you have an accretion disc in there that could potentially be so dense that it is undergoing nuclear reactions.
Even if it is not, it is being frictionally heated and generating its own lights. So, it is stuff waiting to die that’s giving off a lot of the light.
Fraser: The black hole itself – it is totally black. Everything around the black hole – we use that analogy of the water going down the drain, right? There’s only so much water that can go down the drain of your bathtub and the rest of the bathtub water starts to spin around the drain waiting for its turn to go down the drain.
The light that we see from the center of a galaxy is that stuff waiting for its turn to be destroyed by the black hole. Black holes are greedy but they’re just only so greedy.
Pamela: Just to make it clear though the center of our galaxy is bright because there is lots of stuff happily orbiting there.
It is waiting to die because everything dies eventually but it’s not necessarily all going to fall into the center of the supermassive black hole.
Fraser: That’s the difference between an actively feeding black hole and a quiet black hole. I guess the center of galaxies there is just a lot of stuff there. There’s a higher concentration of stars, more stars, brighter.
Pamela: It’s like looking at the downtown district of a city. Why is the downtown area so bright? Well there are lots of people with lots of light.
Fraser: Even if someone goes and turns off the lights at the biggest building downtown you’re still going to see all the other buildings. That’s a good analogy. We’ll go with that one.
Mario Massera asked (one of our favorite questions): “What’s behind a black hole? In other words if you go inside a black hole what will you see on the other side? What is on the other side?”
I guess this is sort of a two part question. I guess on the one hand Mario is asking let’s see, I’m standing and looking at a black hole and I orbit around to the other side, what do I see?
Pamela: The same thing.
Fraser: The same thing.
Pamela: Yeah, ignoring spin black holes are nice symmetric round blobs we don’t know what.
Fraser: [Laughter] Blobs of no light escaping, right.
Pamela: Exactly.
Fraser: So anywhere I want to look at it, what I’ll see is nothing. In fact I’ll see sort of a ring of light being pulled in towards it.
The second question is if I actually hop into the black hole what’s on the other side? Like the black hole is a tunnel and I go through my tunnel and I come out the other side, what do I see?
Pamela: You’re just dead.
Fraser: It’s not a tunnel.
Pamela: No it’s death. The big thing is black holes are just big things. They squish you a lot. They squish you to the point that your atoms are no longer atoms.
They’re some extra state of matter that we haven’t figured out how to define yet. So you’re just dead and a new form of matter.
Fraser: Once again helpful analogies, what’s on the other side of that blender? What’s on the other side of that trash compactor? What’s on the other side of that tree trimmer? [Laughter]
Nothing, horrible death is what’s on the other side of it. You won’t be able to get through the other side of that blender and really consider your surroundings.
All it is is a place where a black hole is just a place where things get squished together. Trash compactor, we’ll go with that. What’s on the other side of that trash compactor?
Pamela: Yeah, the trash compactor that never gets emptied, it just gets bigger.
Fraser: Right. That’s all the time we have for questions this week Pamela so we’ll talk to you again later.
Pamela: Sounds good Fraser.
This transcript is not an exact match to the audio file. It has been edited for clarity. Transcription and editing by Cindy Leonard.