Podcast: Play in new window | Download
Subscribe: RSS
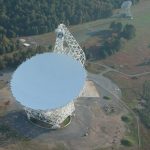
Green Bank Radio Telescope
Astronomers are very resourceful, when it comes to light, they use the whole spectrum – from radio to gamma rays. We see in visible light, but that’s just a tiny portion of the spectrum. Today we’re going to celebrate the other end of the spectrum; the radio end, where photons really stretch out their wavelengths.
|
Shownotes
- Universe Today’s 10th Anniversary
- How big is a nanometer? — Nanooze
- What is Radio Astronomy? — NRAO
- Visual Spectrum — UIUC
- Radio Spectrum — Wiki
- How Radio Telescopes Work — NRAO
- Karl Jansky –– e-notes
- Galactic Center — Max Planck Institute
- Grote Reber — NRAO
- Iridium Satellites
- Iridium Satellite Flares
- Satellite Collision — Universe Today
- Magnetic Fields Shape the Jets Coming From Supermassive Black Holes— Universe Today
- Radio astronomy image gallery from NRAO
- Pulsars — NASA
- Very Large Telescope (VLT)
- ALMA
- What is submillimeter astronomy? — University of Arizona
- Quasars — UTK
- Wow! Signal– Internet Encyclopedia of Science
- Transit Method of detecting extrasolar planets — Kepler Mission page
- Episode 23: Counting Aliens with the Drake Equation
- Episode 129: Interferometry
Transcript: Radio Astronomy
Download the transcript
Fraser Cain: Pamela where are you traveling now?
Dr. Pamela Gay: [Laughter] You know me, I’m never at home. I’m actually down at the Houston Lunar and Planetary Sciences Conference.
Fraser: You may notice that this is the episode for March 23rd; there was no episode last week because we kind of gave up trying to catch up. We’re now caught up and then we can just reset the clock for Monday’s episode and we’ll have the questions show and it should be fine from here on out.
Pamela: But for two and a half years missing one episode, I think we’re doing pretty good.
Fraser: We have a little bit of an announcement which is that today is the ten year anniversary of Universe Today.
Pamela: Wow, congratulations.
Fraser: I started Universe Today on March 23, 1999.
Pamela: You’re an old man of new media.
Fraser: I know. Happy ten years and I’ll try and put a link on Universe Today so people can go back and see the first stuff I ever wrote ten years ago. I know people like it when we keep the chit chat down to a minimum but I just have to tell a story.
My big plan with Universe Today was to learn about space and astronomy because I was always so fascinated with it. I figured I would try and report the news sort of in my spare time and by doing so I would learn about the actual concepts involved.
Then ten years later, I’ve got to say that it worked. If you want to learn a subject, teach it and explain it and you’ll find that you really get to know it quite well inside and out.
Astronomers are very resourceful. When it comes to light they use the whole spectrum from radio to gamma rays. We see invisible light but that is just a tiny portion of the spectrum.
Today we’re going to celebrate the other end of the spectrum, the radio end where photons really stretch out their wavelengths. Let’s just put things in context Pamela. We did a whole episode on the whole electromagnetic spectrum so where are we today?
Pamela: The radio spectrum is the really long wavelengths of light. The stuff that we look at with our eyes is 800ish nanometers, 500ish nanometers.
It depends on if you are a snake or a human. But that’s pretty much where animals are looking at light.
Fraser: Right so peak to peak it is 500 nanometers with a nanometer being a…
Pamela: times to the minus 9 meters.
Fraser: That is a teeny weenie tiny wavelength.
Pamela: When we start talking about radio, we’re talking about stuff that you can actually measure with a meter stick. You’re looking at light that is anywhere from a couple of centimeters long to 20 centimeters long to roughly a meter. You have to use completely different technology as you get to completely different wavelengths of light.
With optical light you can use chemical processes. You can use CCD detectors. You have to have an extremely perfect reflecting surface. One of the unfortunate qualities about light is the surface that you’re using has to be polished to within about one part in 20 of that wavelength.
What that means is if I’m looking at 20 centimeter light, the dish that I’m using to reflect that light can have one centimeter holes in it and the light is not going to care.
If I’m looking at nanometer size light I really can’t have any holes at all. When you start trying to focus radio light you can get sloppy. You can basically use chicken mesh almost.
Fraser: What is the method for capturing radio waves? I think we all understand how visible works, you use a mirror or some kind of lens that focuses the light which is what we would see with our eyeballs.
It is either captured with eyeballs in that you’re just kind of looking through the telescope or it is captured with a CCD camera or with film that we can then look at with our eyeballs. But what is the instrument that can collect a radio wave passing by?
Pamela: A radio telescope actually is in a lot of ways somewhat similar to a reflecting telescope except where a reflecting telescope has a really long tube with a mirror at the end a radio telescope gets rid of the whole tube part and simply has the mirror at the end.
In this case the mirror is just a dish. Anytime you see a satellite dish in somebody’s back yard that’s essentially a radio telescope. It is one however that only looks at things that are being sent down by manmade satellites.
Fraser: Right you’ve got the big dish which I guess is bouncing the radio waves to a detector.
Pamela: That detector you can think of as that mirror that on an optical telescope is up toward the front of the tube. Except in this case instead of having a tube with that secondary mirror the primary focus up at the front of the tube on a spider it instead has a whole set of legs coming off of the dish to suspend it above the dish.
With optical light we’re capable of focusing a whole bunch of light from a swath of the sky onto a detector that has a whole bunch of different pixels on it. We can all at once get a picture where we can make out galaxies, stars and we can make out details. Part of the reason we’re able to do this is because the wavelengths of optical light are so tiny.
Our ability to resolve the sky is related to how many wavelengths of light can fit across your mirror, your detector’s reflecting dish that is capturing all the light. Once you start dealing with radio wavelengths you’re now dealing with really long wavelengths of light. You’re also dealing with a completely different detector technology.
For a variety of different reasons we haven’t really figured out how to make multi-pixel radio detectors. So we have this sensor – a horn – up at the top of the telescope that they focus all of the light onto. With your satellite dish this is the thing that acts as the receiver on your satellite dish. It basically goes, radio signal – no radio signal. Within it, it has a built in oscillator.
When you’re tuning to different channels on your satellite dish which really is just a radio telescope, when you’re tuning to different frequencies you’re actually changing the oscillator inside of that receiver. It is able to filter out different frequencies and say okay I am now going to listen to 20 centimeters, I’m now going to listen to 21 centimeters, and I’m now going to listen to one centimeter.
As it listens to these different wavelengths the strengths of the signals are what we detect as oh I’ve found a radio signal, oh I didn’t.
Fraser: So with a radio it would be as if like with a visible light we would be changing from we were looking at red but now we’re looking at green but instead of seeing all of the pixels that we see, we just see it is either yes there’s red or no there’s nothing.
On off, on off, it’s binary right? I guess with a satellite dish it’s that the frequency is going so fast that you’re getting a television signal. But really it is a binary stream with just offs and ons.
Pamela: There are amplitude modulations and all sorts of different ways that they modulate your television signal and your radio signal. It is the fact that we actually are using radio light to communicate with one another that allowed this form of celestial information to get discovered.
It was Karl Jansky working for Bell Telephone who figured out that objects in the sky are giving off radio signals. He was working on communications equipment for Bell Labs and back in 1931 he found this weird source of interference. He realized that the source of interference peaked about 4 minutes earlier each and every day.
Any of you who are amateur astronomers know that objects in the sky rise about 4 minutes earlier each day. This is because of the difference between sidereal days and sunotic days which is days based off of stars versus days based off of the sun. You have to turn a little bit further to get to the sun.
Fraser: As the Earth is going around the sun we think it takes 24 hours because it takes the sun 24 hours to return to the same position in the sky. The reality is that the Earth actually takes 23 hours and 56 minutes to turn once on its axis.
It is just the fact that we’re also going around the sun and when you add those up it is like 4 minutes every day that gives you back the full 24 hours.
Pamela: It’s that extra bit that got thrown in that allowed you to figure out that whatever this strange 4 minutes earlier every day thing is. It must be located in the sky.
He was able to figure out that the timing of maximum interference actually matched up with the galactic center being high in the sky. It turns out that our galactic center pointed out towards Sagittarius is actually a source of radio light.
Fraser: Was he just like trying to tune into some frequency that they would be using to communicate with?
Pamela: He was just trying to find new ways to send telephone signals using radio light. Instead he discovered the galactic center. At least he discovered it in the new color of light. This was so new that there were no astronomical telescopes dedicated to radio work.
We had lots of optical systems, we had reflectors, refractors. It was the 1930s it was the golden age of getting started on building the great big telescopes. But radio didn’t exist.
The very first radio work was actually done by Grote Reber who was just an amateur radio astronomer in my current home state of Illinois. He worked in his back yard and when he submitted his first paper for publication, the optical astronomer who read it couldn’t really fathom what he was reading so he went out to this guy’s back yard to make sure that the data was real and see what was going on.
After getting his head wrapped around the wow the universe exists in radio as well idea, this led to in the 1950s the National Radio Astronomy Observatory was built. This was the first national observatory because it was recognized that no one institution could really build the technology that was needed for radio astronomy.
They didn’t steal but they basically built on a lot of the technology that was built during World War II for radar work. The National Radio Astronomy Observatory was the first national observatory and it took military technology and turned it over to astronomy to help us understand the universe a little bit better.
Fraser: What then is radio good for? Why is it not as good, if you only see one color at a time why wouldn’t we to just look in beautiful visible light with all of its wonderful pixels?
Pamela: There are different things that you can learn from different colors. In optical light we’re seeing a lot of hot things. We’re seeing the transitions of atoms like hydrogen and neon just like the transitions that you get in science. We’re seeing basically atomic lines. We’re seeing gas that’s in thousands of degrees temperature.
Most of the universe isn’t thousands of degrees. Most of the material in the universe isn’t necessarily in really hot stars. To see this colder material we have to instead start looking for much more cool thermal reactions that occur down at a few degrees Kelvin.
One of the neat things is just studying how the hydrogen atom exists in a lot of different forms. You can look out and you can see ionized hydrogen. You can look out and you can see plain old everyday neutral hydrogen. This is where the 21 centimeter line becomes so important.
Hydrogen by itself is responsible for a lot of interesting atomic transitions that we see in stars. The bomber series gets talked about a lot in astronomy classes. Completely cold hydrogen, so cold that none of the electrons are at all interested in moving has a small probability that an electron in the lowest energy level just hanging out doing its orbital thing will flip.
Protons and electrons both have what is called spin. Depending on if the spin of the proton and the electron are both point up or if one is pointed up and the other is pointed down, you have slightly different energies. The energy needed to go from spin parallel to spin to one up one down, that energy corresponds to 21 centimeter light.
Fraser: This is a situation that the electron has flipped.
Pamela: It gives off just this little amount of energy.
Fraser: Right, this 21 centimeter photon. That tells you that you’re seeing…
Pamela: Cold gas.
Fraser: I guess I’m wondering then what is the methodology that they would use to look at this. Did they take the telescope and look at one little spot on the sky and say yes I’m receiving [Laughter] 21 centimeter light and they move it just a tiny little bit over and go yes I’m receiving so they maybe kind of try to trace out the borders of it?
Pamela: Pretty much but what you end up with actually is a beam on the sky. As you scroll the beam across the sky if it is dominated by lots of neutral hydrogen gas then you pick up lots of signal. If it is half on half off you get less signal. So as you scan across you’re getting a smeared out ugly signal.
Using computers you can put that together to build up a picture. You can imagine that instead of taking your pretty 5 mega pixel image all at once you instead have a single pixel detector that you slowly scan up and down, up and down, up and down until you’re able to build up that mega pixel image.
Fraser: There are a couple of advantages to radio astronomy.
Pamela: You can see through the clouds.
Fraser: You can see through clouds, you can see the day, right? Radio astronomy doesn’t care whether it is day or night, cloudy or sunny it just works. Choose your own schedule.
Pamela: At the same time though radio astronomy does work in the same wavelength that telecommunications wants to work at. There are different parts of the planet that are supposedly – and I say supposedly because of the annoying Iridium satellite system which is really pretty to look at – but gives off light in colors it shouldn’t.
Fraser: Especially when they collide with other satellites.
Pamela: Well they do that too. [Laughter] But there are different places that are protected zones that the colors that are most interesting for astronomical purposes are protected. We can go out and look for certain molecules. We can go out and we can look for carbon monoxide.
One of the annoying things is molecular hydrogen has no molecular lines. We can’t see it in any way whatsoever but we at least have carbon monoxide gas that we can go out and look for. There are carbon sulfide gases. There are all sorts of different molecules in space. We found water, formaldehyde, we found methane.
Fraser: Alcohol.
Pamela: All of these different – yeah, alcohol. All of these different molecules are out there rotating and it is from what we call rotational transitions. They give off different amounts of energy depending on how they are rotating, what rate they are rotating at. These different rotational transitions allow us to see the lines using radio telescopes.
Fraser: I guess it takes some math to figure out if you’re seeing alcohol then you’re going to be seeing radio waves coming off of it at this wavelength?
Pamela: Unfortunately this is all very ugly quantum mechanics. When you’re first learning quantum mechanics you start off with here’s the hydrogen atom. You have a proton, you have an electron life is good. You then learn how to handle the helium atom and you cry.
Fraser: We should really do that show. [Laughter] Not the crying one, the one before. Let’s save the crying one for later. We’ll do the easy hydrogen.
Pamela: Then you get to more and more complex atoms and about the point that you think that you’ve started to understand quantum mechanics someone throws a molecule at you. There are all sorts of crazy notations from Dirac notation to Krupps-Gordon to 3J where you swear the mathematicians just started making stuff up.
But it works and what’s amazing about all of these different complicated scenarios where we’ve actually been able to make it so that you can fit what you’re doing on a piece of paper we had to come up with symbols to represent complicated equations.
By working through mathematics using this language of symbols we’re able to accurately predict where is OH going to have lines. Where is Salt, NaCl going to have lines? We’re able to find in comets, in cold gas and in a whole variety of different places all of the different molecules. They exist in space and their signatures are exactly where we expect them to be.
Fraser: Can you give me some more kinds of uses for radio astronomy? You mentioned you’re looking for large blobs of cold hydrogen gas. You’re looking for crazy molecules that maybe [Laughter] we expected were only on Earth but in fact they’re out there too. What are some other reasons that you would want to look at something in radio light?
Pamela: Other things that you can do with radio light are you can study high energy jets coming off of super massive black holes. That sounds kind of counterintuitive.
Fraser: Would I use my x-ray telescope for that?
Pamela: Well you can do that too but that you have to put into orbit. That’s expensive. One of the convenient things about super massive black holes is they have nice powerful magnetic fields.
If you have an electron moving the correct way through a powerful magnetic field it is going to end up going in little tiny spirals. So, instead of shooting off at a diagonal it is going to get caught by the magnetic field and it is going to end up going in basically a ringlet pattern shooting through the magnetic field.
As the electron spirals it has to constantly be accelerating. Accelerating means you’re either changing your speed or you’re changing your direction. The electrons are losing energy over distance but in general they’re over a short couple of cycles around moving at constant speed.
Since they’re spiraling that means they’re constantly changing direction which means they’re constantly accelerating and that means they have to give off energy somehow. They give off their energy with synchrotron radiation that energy comes off in radio. It’s not that much energy that they’re losing as they’re going in these tightly wound spirals away from the super massive black holes.
However, it is enough that when we look out at some of the most amazing radio galaxies we see these huge jets that when you take a picture that can encompass all of both jets the main part of the galaxy in the center of the image is only maybe a few hundred pixels across while the jets themselves are thousands of pixels in length.
So we’re able to see an entirely different type of physics that we wouldn’t know about without radio or x-ray. In the radio you can start to get to the fine structure of the electrons interacting with the material between galaxies. You can see how they go from very tightly bound in close to the center of the galaxy to spreading out.
We can even see how over time galaxies have rotated such that just like when you move a hose with the water coming out you leave an arc of water behind. These moving galaxies leave arcs of jets behind as they move.
Fraser: This technique comes in wherever you’ve got electrons moving through powerful magnetic fields. You’re going to get this tiny little bit of energy loss which is going to be ejected as photons.
You’re going to be able to detect it. I guess they come in very useful for seeing pulsars?
Pamela: We have pulsars in another place that you have electrons moving through magnetic fields. They are also useful for detecting star formation. Radio waves pass through really thick dust and gas. Optical light doesn’t do that.
We can look into star forming regions and see stars being born and then we can look at pulsars and see stars that have died. So you can get at both the tail ends of a star’s life by using radio light.
Fraser: In the same way that the cosmic microwave radiation was once visible light, do you get situations where certain kinds of light has been stretched out say from infrared all the way out into the radio spectrum like further out?
Pamela: I’m sure there are cases of interesting infrared light getting stretched out into the millimeter wavelengths stretched out to the centimeter wavelengths but I have to admit that is not something that I’ve looked into.
Fraser: Zing.
Pamela: Yeah it happens, you get me now and then and you just got me pretty good. I can imagine some of the interesting submillimeter stuff. Submillimeter is really hard to work with because to get a really good image you have to worry about the water in the atmosphere so you have to build your telescope someplace very high like the Atacoma desert where they’ve built the VLT and where they’re currently building ALMA.
Fraser: There is an episode on that coming up on submillimeter.
Pamela: You also have to have much more perfect dishes. If you’re trying to look at the submillimeter signal from something that is extremely far away you might be able to get that hard to detect signal over into the radio light.
The problem is its mass is so far away that it is really really faint and now you have a different problem to deal with.
Fraser: I guess that was one of my next questions is what about distance? I know that Hubble and so on can see galaxies that are billions and billions of light years away.
Does the same thing work with radio waves? Can you still see right out to the edges of the observable universe assuming you’ve got a bright enough source? [Laughter]
Pamela: Right, so we haven’t found objects that are at the same very beginning of the universe stars forming for the first time red shifts in radio. What we have found is lots of quasars that are at very large distances.
There are galaxies with very active super massive black holes in their center that are extremely loud in radio. It’s also an orientation issue. If you have a galaxy that you’re looking edge-on so say it’s a flat disc galaxy like Andromeda or like our own Milky Way and you’re looking along the edge of the disc all of that material is going to take and make the radio light have to penetrate through more stuff.
While dust is transparent to radio light you can still block a signal from the center given a galaxy between you and the center of the galaxy. If instead you rotate that galaxy 90 degrees so you’re looking straight down on the super massive black hole in the center you first of all if it is active get the jets coming out towards you. You’re looking straight in on the jets. You’re getting the synchrotron radiation pointed right at you, which is convenient.
You also do not have to worry about what the disc of the galaxy itself might be blocking. Orientation matters but there are lots and lots of radio loud quasars that we’ve detected.
Fraser: I think it is one of the last really good uses of radio telescopes are searching for extraterrestrial civilizations, right? [Laughter] We could do an episode on that.
Pamela: There was actually the anniversary this week. There was a detection of what we call the WOW signal which was this one random signal in a bit of radio data that was just all of a sudden very loud. It looked like it could be something very interesting and then it didn’t repeat itself which has always been a big piece of sadness.
Fraser: Nobody even knows what it was.
Pamela: But there are lots of different places that are with varying amounts of dedication looking for signals from extraterrestrials. One of the neat ways to do it is to purposely look out along the direction of if you were on this band in the sky you would be able to see Jupiter or other planets in our solar system transiting across the surface of the sun.
We’re looking for the people who could see us to see if they’re sending signals to us. That’s the best way we have of potentially detecting them is detecting a beam signal that was sent purposely toward us.
Fraser: This is the technique that we use to find extrasolar planets where we can see the planets transiting across the star we would assume that those aliens would be looking for us in the same way.
They would see the Earth transit or Jupiter transit across the sun and so they would have to be stars that are in a very thin ring around the sun, in a very tight band in the sky so we could look there.
This was a fairly recent article. It is a great idea.
Pamela: We talked about it I believe in our Frank Drake equation episode so you can go back and hear a lot more about this idea.
Fraser: I think we mentioned we don’t want to kind of go over it in great detail but I think we talked about it last week about interferometry and how radio telescopes work really well to link up many of them together.
Pamela: One of the neat things is by having multiple telescopes linked together it also allows you to start building pictures in a completely different way.
But that is something that we’ll talk about more when we talk about submillimeter next week.
Fraser: Okay, well we’ll talk to you for the next question show and in a week for the submillimeter.