Podcast: Play in new window | Download
Subscribe: RSS
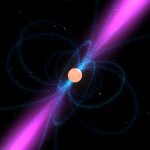
Artist's illustration of a pulsar
Imagine an object with the mass of the Sun, crushed down to the size of Manhattan. Now set that object spinning hundreds of times a second, blasting out powerful beams of radiation like a lighthouse. That’s a pulsar, one of the most exotic objects in the Universe.
|
Shownotes
- Pulsar Tutorial — Science@NASA
- Pulsars –– Goddard SFC
- Pulsars — UTK
- Neutron Stars VS. Pulsars
- Conservation of Angular Momentum — UTK
- Fast spinning pulsars (millisecond pulsars) –NRAO
- 1974 Nobel Prize in Physics to Antony Hewish for the discovery of pulsars
- Jocelyn Bell Burnell — UCLA
- 1993 Nobel Prize in Physics to Russell Hulse and Joseph Taylor for discovery of binary pulsars
- Gravitational Radiation — DrPhysics
- Crab Nebula Pulsar — SolStation
- Paper: Why Study Pulsars Optically; A. Shearer & A. Golden
- Planet Hunting — How Stuff Works
- Discovery of PSR1257+12 by Alexander Wolszczam and Dale Frail — Internet Ency. of Science
- Pulsar Planet Star System — Extrasolar Vision
- Pulsar 4U 0142+61 with protoplanetary disk — Spitzer
- Fastest Spinning Pulsar IGR J00291+5934 — Universe Today
- Magnetar — Wiki
- Magnetar that “blinded” satellites in 2004 — Space.com
Transcript: Pulsars
Download the transcriptFraser: Astronomy Cast Episode 158 for Monday October 5, 2009: Pulsars. Welcome to Astronomy Cast, our weekly facts-based journey through the Cosmos, where we help you understand not only what we know, but how we know what we know. My name is Fraser Cain, I’m the publisher of Universe Today, and with me is Dr. Pamela Gay, a professor at Southern Illinois University Edwardsville. Hey, Pamela.
Pamela: Hey Fraser, how’s it going?
Fraser: Good. Pulsars! So, imagine an object with the mass of the sun crushed down to the size of Manhattan. Now, set that object spinning…hundreds of times per second… blasting out powerful beams of radiation like a lighthouse. That’s a pulsar…one of the most exotic objects in the universe. Alright, Pamela, so maybe you can give another equally gripping description of what a pulsar is. If we could see a pulsar, what would we see?
Pamela: The coolest thing about this is if you took a thimbleful of the surface and looked at just that one thimbleful of the surface, it would weigh a hundred million tons, and it would be going around and around something the circumference of Manhattan roughly… something about 12 miles in diameter…as fast as 1000 times per second. It’s kind of extreme!
Fraser: Yeah…yeah. Well, then I think we need to go back first and hear the story…just where do these things come from?
Pamela: Well, when a mommy and daddy star get together…
Fraser: So, where do we get a pulsar from?
Pamela: There’s actually 2 different general species of pulsars. In one case, you take a big ol’ star and you let it die violently as a supernova. In some cases you end up left behind this core of the star that contains all of its angular momentum. You take all of that rotational energy, keep it…at least keep most of it…and set it loose rotating something that’s way smaller. So this is the equivalent of taking your ice skater who has her arms spread out straight and not only having her wrap her arms around her body, but have her body suddenly become smaller than a piece of spaghetti around. So not only does the speed increase a little, it increases a whole lot with that conservation of angular momentum.
Fraser: So a pulsar was once a star, it detonated as a supernova, and you’re just left with some super-dense core that remains, but it still has all of the spinning that it had when it was a big star.
Pamela: In the case of pulsars, they also have a magnetic field. Magnetic fields are one of those things that when you try to explain them, people get confused and it’s hard. But they have these really powerful magnetic fields that aren’t fully understood, that aren’t lined up with the rotational axis of the star. This is the same thing that we have here on Earth…the Earth’s north pole and south pole according to rotation and according to your compass aren’t the same place.
Fraser: Now you said two ways to get them…one was an exploding star…
Pamela: And the other is that you wait a while and that exploding star will…you had a nice fast rotating pulsar…it’s going to slow down, slow down, slow down. But then if you add mass to its surface, if you put it into a system where it either has winds from a nearby companion star blowing on it and dumping matter onto its surface, or where it’s stripping matter off of a nearby companion star through gravitational pull and filling of what we call the Roche Lobe, that extra material falling onto the neutron star can also spin it back up, get it moving fast yet again…a recycled pulsar is one way they get referred to. These are the x-ray pulsars. We have the high-speed pulsars like the one inside the Crab Nebula that are rotating away because they’re young, and that’s just what young dead neutron stars do is they rotate quickly. But then we also have the recycled ones that have lived a second life that are now also emitting in the x-ray that are rotating quickly because of extra matter that has gotten dumped onto them.
Fraser: Right. And we’ve done a few shows on this already that a pulsar is — for all intents and purposes — a neutron star, but it has some different behavior from a neutron star, which is what makes it a pulsar. So then, what’s it made out of?
Pamela: Well, neutron stars are made of neutrons, basically. This is where you have material that is so dense that the electrons and protons no longer can hold themselves apart. You have white dwarf stars, which are where you pack as many protons and electrons together as you possibly can. Well, if you try to pack them a little bit closer together, if you increase the mass to the point that the electrons and protons can no longer keep themselves apart, then they smoosh together and become neutrons. Neutrons are only stable in these extremely dense environments, or when they’re down in the centers of atoms. Now, outside of this neutron star there’s roughly a mile-thick crust of other stuff. Now, what’s cool about neutron stars is occasionally they glitch. So in general, one of the awesome things about pulsars is that they’re out there rotating, rotating, rotating, and the fastest ones…they’ll rotate 500 times a second, 600 times a second, 700 times a second…most of them aren’t that fast. But in general, all of them are so consistent that they make some of the best clocks in the entire universe. We can look out and be looking at one of these high-speed pulsars and slight variations on the surface of the planet Earth suddenly become measurable as timing changes in the pulsar. One of the neatest things I learned in grad school is they actually have to worry about the tides on mountains when they’re looking at pulsars….how your observatory moves toward and away from the pulsar as the moon goes around and around the planet Earth. They actually have to worry about that and that’s really, really cool.
Fraser: So hold on…they’re worrying about your motion towards and away from the pulsar?
Pamela: Yes, you can actually measure that as timing differences over the period of watching this pulsar over and over and over and over again. So, as you take several days worth of observations of a given pulsar, you can actually start to see variations in when the pulses arrive that are caused by your own position on the planet relative to the pulsar changing due to the tides on the earth.
Fraser: Right, OK, so I’d like to sort of figure out this distinction, then. So, if I had a great big star with a lot of mass, but it wasn’t rotating… it was just kind of…somehow the angular momentum had all worked out and it just wasn’t turning very quickly. Then it blew up as a supernova and then collapsed down as a neutron star and maybe it’s turning… like a star… once every couple of days, say. We would not see that at all as a pulsar, right?
Pamela: No. No. In fact, there are believed to be nice happy little neutron stars out there that are rotating probably several times a day that we don’t see as pulsars just because the variations aren’t such that we can see them. As the star’s rotation slows down, the amount of energy that’s getting beamed out through the magnetic field goes down.
Fraser: Right, so it still has very powerful magnetic fields, but it’s not being bled away in the way that a pulsar is. So, then where would we kind of draw that line? When does it turn into a pulsar? When do we start seeing all the action?
Pamela: When we look out, we see pulsars going at all different speeds where they have a pulse every 1.4 milliseconds out to every 8.5 seconds. Now, we really stop seeing them as pulsars once they get much slower than 1 rotation every 10 seconds or so, and what’s happening is each of these different types of pulsars has a slightly different energy generation mechanism. We have the radio pulsars — these are the first ones that were discovered. They’re powered through the rotation as they rotate around the radiating energy out of what essentially look like lighthouse beams where the magnetic fields line up with rotational axis of the star. Then we also have the X-ray pulsars, which are really powered by the material that’s falling in onto them. Now, with these two different types of stars, part of the reason that we’re able to identify them is that we look for these specific every-few-second rotation beats to way-less-a-second rotation beats and the type of energy that comes out is related to how fast they are rotating. We’re also able to see the X-ray pulsars because, well, they show up on X-ray, which is always a nice, easy convenient way to find high-energy objects because the X-ray sky isn’t all that loud. Once they start slowing down past that, they’re just not beaming the energy out as much, and so then the neutron stars…they start being identified more as just being these little, hot critters glowing faintly on the sky because they’re so tiny.
Fraser: Now, you mention in sort of a throw-off comment there that they’re slowing down, that they’re giving off energy, but can you sort of describe that mechanism in more detail? How are they losing energy?
Pamela: Well, as they rotate, light leaving them, it’s carrying away light, energy, mass – it’s all different faces of the exact same thing. And so, as this light gets radiated away, it’s changing the star, and the energy goes away, the angular momentum drops and you have a star that’s slowing down, and slowing down, and slowing down over time. Now, it’s not a huge effect. Something that is rotating once per second is, over the course of a century, going to have lost the smallest fraction of a second off it’s rotational period, so this isn’t the type of thing that we generally see very much as we watch these stars evolve through time, but they are slowing down.
Fraser: So, back to our “Einsteinian” calculations: mass and energy being equivalent, you’re saying you take the total mass of the neutron star, and then you include the energy, the rotational energy of the star, as one whole collection of mass and energy, and that defines how fast it’s going to spin. I’m sure there’s a real easy calculation – you just punch it all in — but that defines the speed of its rotation. And then it is turning through radio waves, or in the case of X-rays, it’s emitting that radiation and that radiation is just pulling out of that entire equation, so it has less mass and energy and so therefore, it doesn’t have to rotate as quickly.
Pamela: Right, so as you change the angular momentum of the system, the rotation rate changes with it, and the best way to change something’s angular momentum is to remove some energy from the system and beaming light is a great way to remove energy. Now, what’s cool is there’s actually been a couple of different Nobel Prizes given for discoveries in pulsars. The first Nobel Prize went to just, well, discovering pulsars existed, and it was actually kind of controversial. Back in 1967, Jocelyn Bell Burnell (who’s now at Oxford), and Antony Hewish discovered the very first pulsar while looking out in the radio light, and it was only Antony Hewish who received the Nobel Prize, and Jocelyn Bell Burnell was kind of left out of that particular award, but nonetheless, it was a rather dramatic discovery. We didn’t know stars could do this. Well then, almost a decade later, in 1974, it was another team discovery, and in both cases this was an advisor and a graduate student doing the work. In the second case, it was Joe Taylor and Russell Hulse who were out studying pulsars and they found a pulsar in a binary system with a second neutron star, and these two stars were orbiting each other in about 8 hours. Now, one of the things that got predicted by Einstein in general relativity was that these extremely high-mass systems should be radiating away gravitational radiation, radiating away gravitational waves, and this would cause the entire orbital system to lose energy. Now, they were actually able to see that gravitational radiation in terms of evolution of the orbit of these two neutron stars over time, so we’ve been able to test relativity and award a number of Nobel Prizes thanks to these little tiny stars that, like you’ve pointed out, they are about the size of Manhattan.
Fraser: And I think this is where some of the really useful things from pulsars come from. As you said, they’re so precise you could just record a pulsar over the course of 100 years, it would slow down a tiny little bit, but essentially if it’s 700 a second, you’re going to get these little radio pulses from the pulsar 700 a second, and so from that you can measure things that it’s interacting with with tremendous precision [sic].
Pamela: And what’s amazing is, in some cases, we’ve been able to optically detect that these pulsations are happening. Now, this is a lot harder – it’s not like we have lots and lots of high resolution, very sensitive cameras that are geared up to be looking for this high speed of pulsation, but the pulsar inside the Crab Nebula – we can actually see it in optical light doing its pulsar thing. It’s one of a very small number of systems that are in a paper by Shearer and Golden. We really only know of six of these, but yeah, they’re pulsating in optical light as well as in the radio light. They’re amazing little systems, and they’re not extremely common, but we are finding new ways to find them thanks to using the X-ray observatories, thanks to going out and doing new radio surveys of the sky, so we’re constantly finding new ones, and we’re constantly finding new ways to use them. Because they are so precise, we can use them to measure things that I don’t think people originally thought about — like little tiny planets.
Fraser: Right, so that’s what I wanted to talk about. What are some of the things, then, that you can use a pulsar to study? So, you’re saying planets — we’ve talked about that a bit, that planets have been discovered — so, what’s the mechanism that they use to discover planets around a pulsar?
Pamela: Well, with normal stars we’d look for planets either by looking for the planet to pass in front of the star and cause its light to get dimmer, which is really, really hard to do, but you can do it with a 4-inch telescope that’s really well-calibrated. But the more common way to go out and look for planets, is to look for how the planets tug gravitationally on the star they’re orbiting. We can measure this with the Doppler shifts of the star, where we can actually see the stars wobble about their passage through the sky as they get tugged to and fro by the planet that’s going around them. Well, with pulsar planets, we aren’t exactly looking at the spectra, but what we can do is look at the shifting in the arrival times of the pulses that are coming toward us. And back in 1992, Alexander Wolszczan and Dale Frail discovered the first multi-planet planetary system, and it wasn’t just the first multi-planet planetary system, it was flat-out the first planets ever discovered outside of our own solar system, and they discovered it by looking at timing issues in a pulsar that was ever-so-creatively named PSR 1257+12. What they found was the system had very complicated…light arriving too soon, light arriving too late…and the only way to explain this dance in the pulse timings was to invoke multiple planets.
Fraser: So, with the radial velocity method of planet finding you’ve got that Doppler shift back and forth, so they were actually able to not just see the Doppler shift from one planet, but it was almost like a bit of a dance, right? So, the timing was off a little here, a little there, a little here…and they were able to then reverse engineer how many planets, what mass they had, and what impact they were having on the rotational speed of the…or on the sort of, I guess, the radial velocity of the pulsar. That’s crazy!
Pamela: And really it was just the tug-of-war changed, in some ways, the distance from the pulsar to the planet Earth, and with those little tiny changes in pulsar position, we saw changes in when the light arrived here. And what was amazing about these first planets that were found is they were only a few times the size of the Earth. The first two that were found were 4.3 and 3.9 times the size of the Earth, and we’re still struggling to find things this small using all of the other techniques that we know of. Now, pulsar planets aren’t something we discover every day. We’ve found 300-ish planets around other stars at this point that are nice, normal, healthy stars. But when it comes to confirmed pulsar planets, we really only have five, and they’re going around two different pulsars, so the universe isn’t filled with pulsar planets, but what’s neat is the pulsar planets — they offer us a different way of potentially getting at planets. One thing that’s been discovered is there’s what looks like a normal everyday proto-planetary disk around one particular pulsar: 4U 142+61. The disk of material around this little object – it was created when the star blew itself to bits, and so it appears that stars can recycle themselves and create their own lifeless, new planetary system around the remnant star they leave behind.
Fraser: Yeah, I mean, it sure wouldn’t be a wonderful place to live without a real, you know, life-giving star, but it shows that the whole process of planets coming back together again is all still…that still works, even though the star is dead. I guess it’s still a source of gravity, and why not?
Pamela: And at the end of the day it’s all about the gravity.
Fraser: Yeah, and so we’ve talked about pulsars have been used as a method for working out gravitational waves and confirming relativity. Pulsars have been used to find planets and probe planetary formation in thought-dead star systems. So, what else are pulsars good for?
Pamela: Well, in studying the ways that they misbehave, we can also start to understand the insides of these extremely dense objects a little better. As we watch them over time, occasionally we see these little glitches in the timing. So, if you watch cycle to cycle to cycle, they appear to be the most amazing atomic clocks ever, but then they sometimes hiccup — and not all of them, just some of them — and it took a while to figure out what was going on, and it seems that in some cases, over time, the insides of the star will rearrange slightly. This is thought to maybe have something to do with the semi-conducting properties of the material inside the star changing over time. And as the insides of the star rearrange, it’s sort of like that ice skater taking her arms and going from having them crossed one way to having them crossed another. When you change the distribution of material, you change the moment of inertia of the star – ever so slightly — and this can create a glitch in the period of rotation, and so we can see how stars rearrange themselves by looking at how the stars’ timings appear to hiccup now and then.
Fraser: And I guess we would be able to see if a chunk of matter falls onto a pulsar, right? Because it would change the overall momentum of the system…
Pamela: Right, and so far we haven’t seen this in the X-ray ones that we look at. We do see these X-ray systems where they do constantly have matter falling onto them, but so far we haven’t seen this sudden “Oh, it just ate a planet!” change in period that you might get from a sudden ingestion of one chunk of matter, rather than the slow ingestion of a continuous stream of matter.
Fraser: Yeah, they’re sucking some other star through a straw.
Pamela: Right. Exactly, exactly…
Fraser: OK, so what else are they good for?
Pamela: Well, it’s just cool knowing that our universe has these high-energy lighthouses out there, and can give us this sense of, well, what is the frequency at which you end up with these high energy binary systems in different places. So, as we look out at globular clusters, and we see these X-ray pulsars, theses recycled systems, where the pulsar is getting sped up from eating material off of a companion, either just through sucking it straight out, “sucking it through a straw,” as you put it, or by ingesting the stellar winds. We can look at these systems and say, “OK, this is the frequency at which binaries either get born, get created by one star grabbing the other.” It’s neat to be able to understand how stars exist, and neutron stars, they aren’t exactly giving off a lot of light, but pulsars, because they’re so weird, allow us to pinpoint them in distant globular clusters and understand the properties of stellar populations in these distant systems.
Fraser: And I think we talked a couple of episodes ago that one of the fastest, or the fastest star in our galaxy is a pulsar.
Pamela: Right, it’s a neutron star that got ejected during an asymmetric supernova explosion. And we can’t fully explain how that happens, but if you explode something in just the right crazy sort of way, you can end up sending a neutron star not just flying through the galaxy, but flying out of the galaxy.
Fraser: Right, the supernova went off more like a rocket than an explosion that blew it out of nowhere.
Pamela: Yes.
Fraser: Well, that is really cool! Now, are there any…what would you say are like the big outstanding mysteries about pulsars? I guess you hinted a bit as to what is the actual configuration of their structure.
Pamela: And we know neutron stars are just part of a phase of evolution that have these regular pulsars that are rotationally-driven, that have these X-ray pulsars that come from ingesting matter, and then there’s this other mysterious critter known as a “magnitar,” which is an extremely magnetic object, that isn’t pulsating, but periodically gives off bursts of X-ray and gamma ray light. And trying to put all these pieces together of how neutron stars, which, on one hand are extremely simplistic objects…they are just giant balls of neutrons. Well, how is it that these simplistic objects have such powerful magnetic fields? How is it that they transition and can be all three of these different faces? And, can one object pass through all three of these different stages? We’re still figuring out how to put all these other pieces together.
Fraser: Right. So, is it almost like human life, right? You start out as a baby, then you’re a teenager, then you’re middle-aged, and so does this class of object, I mean they’re all just a ball of neutrons, but do they all start out as pulsars, and then turn into magnitars, and then spend the rest of their lives as neutron stars? Is there some different combination, or are they just, depending on the rotation of the star beforehand, or how the mass of it was, or what its magnetic field was? Does that define what kind of an object, or how it behaves? Even though at the end of the day it’s just a ball of neutrons.
Pamela: And trying to understand is complicated by the fact that these are faint, that none of them are nearby. And it’s also made more interesting because they’re some of the most dangerous objects in the galaxy. One of these that went off in 2004 – a magnitar on the other side of the center of the galaxy from us – was able to temporarily blind some of the satellites orbiting the earth by blasting their cameras with so much energy that they had to be basically turned off and on a few different times to clear out all the extra light. So, they’re interesting; they’re fascinating. They look like they should be simple, but they’re not. And they do so many different interesting things that we can apply in so many different ways — from finding planets to better understanding the behavior of our own planet — that they’re definitely worth studying.
Fraser: Cool! Alright, well, thanks Pamela.
Pamela: It’s been my pleasure, Fraser. I’ll talk to you next week.
This transcript is not an exact match to the audio file. It has been edited for clarity.